The age of Homo naledi and associated sediments in the Rising Star Cave, South Africa
- Cited 50
- Views 19,085
- Annotations
Cite
as: eLife 2017;6:e24231
doi: 10.7554/eLife.24231
- Article
- Figures and data
- Side by side
- Jump to
Abstract
New ages for flowstone, sediments and fossil
bones from the Dinaledi Chamber are presented. We combined optically
stimulated luminescence dating of sediments with U-Th and palaeomagnetic
analyses of flowstones to establish that all sediments containing Homo naledi
fossils can be allocated to a single stratigraphic entity (sub-unit
3b), interpreted to be deposited between 236 ka and 414 ka. This result
has been confirmed independently by dating three H. naledi teeth
with combined U-series and electron spin resonance (US-ESR) dating. Two
dating scenarios for the fossils were tested by varying the assumed
levels of 222Rn loss in the encasing sediments: a maximum age
scenario provides an average age for the two least altered fossil teeth
of 253 +82/–70 ka, whilst a minimum age scenario yields an average age
of 200 +70/–61 ka. We consider the maximum age scenario to more closely
reflect conditions in the cave, and therefore, the true age of the
fossils. By combining the US-ESR maximum age estimate obtained from the
teeth, with the U-Th age for the oldest flowstone overlying Homo naledi fossils, we have constrained the depositional age of Homo naledi to a period between 236 ka and 335 ka. These age results demonstrate that a morphologically primitive hominin, Homo naledi, survived into the later parts of the Pleistocene in Africa, and indicate a much younger age for the Homo naledi fossils than have previously been hypothesized based on their morphology.
https://doi.org/10.7554/eLife.24231.001
eLife digest
Species of ancient humans and the extinct
relatives of our ancestors are typically described from a limited number
of fossils. However, this was not the case with Homo naledi.
More than 1500 fossils representing at least 15 individuals of this
species were unearthed from the Rising Star cave system in South Africa
between 2013 and 2014. Found deep underground in the Dinaledi Chamber,
the H. naledi fossils are the largest collection of a single species of an ancient human-relative discovered in Africa.
After the discovery was reported, a number of
questions still remained. Not least among these questions was: how old
were the fossils? The material was undated, and predictions ranged from
anywhere between 2 million years old and 100,000 years old. H. naledi
shared several traits with the most primitive of our ancient relatives,
including its small brain. As a result, many scientists guessed that H. naledi was an old species in our family tree, and possibly one of the earliest species to evolve in the genus Homo.
Now, Dirks et al. – who include many of the researchers who were involved in the discovery of H. naledi
– report that the fossils are most likely between 236,000 and 335,000
years old. These dates are based on measuring the concentration of
radioactive elements, and the damage caused by these elements (which
accumulates over time), in three fossilized teeth, plus surrounding rock
and sediments from the cave chamber. Importantly, the most crucial
tests were carried out at independent laboratories around the world, and
the scientists conducted the tests without knowing the results of the
other laboratories. Dirks et al. took these extra steps to make sure
that the results obtained were reproducible and unbiased.
The estimated dates are much more recent than many had predicted, and mean that H. naledi
was alive at the same time as the earliest members of our own species –
which most likely evolved between 300,000 and 200,000 years ago. These
new findings demonstrate why it can be unwise to try to predict the age
of a fossil based only on its appearance, and emphasize the importance
of dating specimens via independent tests. Finally in two related
reports, Berger et al. suggest how a primitive-looking species like H. naledi survived more recently than many would have predicted, while Hawks et al. describe the discovery of more H. naledi fossils from a separate chamber in the same cave system.
https://doi.org/10.7554/eLife.24231.002
Introduction
The fossil assemblage attributed to Homo naledi from the Rising Star Cave in the Cradle of Humankind, UNESCO World Heritage Area, South Africa (CoH) (Berger et al., 2015), represents one of the richest and most unusual taphonomic assemblages yet discovered in the hominin fossil record (Dirks et al., 2015).
The remains are exceptionally well preserved and represent the largest
collection of fossils from a single primitive hominin species ever
discovered in Africa. The H. naledi fossils occur without a
direct association with non-hominin macrofossil remains, and are found
deep inside the difficult to access U.W.101-Dinaledi Chamber (Dirks et al., 2015).
The Dinaledi Chamber is characterised by a sedimentary environment that
is geochemically and sedimentologically distinct from the rest of the
Rising Star Cave (Dirks et al., 2015),
and the fossiliferous deposit it contains is profoundly different from
other known hominin-bearing cave assemblages in the CoH (e.g., Reynolds and Kibii, 2011; Dirks et al., 2010; Pickering et al., 2011a; Dirks and Berger, 2013; Bruxelles et al., 2014).
The fossils occur as a dense bone accumulation in mostly unconsolidated
muddy sediment that largely originated from within the cave through
weathering of the dolomite host rock (Dirks et al., 2015). The fossils have not been dated until now.
In this paper we present results of uranium-thorium
(U-Th) disequilibrium, electron spin resonance (ESR), radiocarbon, and
optically stimulated luminescence (OSL) dating in combination with
palaeomagnetic analyses, to provide ages for the fossils and surrounding
deposits in the Dinaledi Chamber, and build upon the geological context
described in Dirks et al. (2015).
Dates acquired via U-Th and ESR techniques were obtained using a double
blind approach for each technique to ensure robust, reproducible
results, with each laboratory using their own analytical and
computational approach. Approaches taken by each laboratory that
contributed to this paper are described in detail in the methodology
section.
The age of the hominins in the Dinaledi Chamber has
implications for our understanding of the mode and tempo of the
morphological evolution of hominins (Hawks and Berger, 2016),
raising questions about evolutionary stasis and the role of refugia.
The results challenge our ability to associate given hominin species to
specific cultures and behaviours in the past. These issues are discussed
in greater detail in an accompanying paper (Berger et al., 2017).
Geological setting
The caves in the Cradle of Humankind (CoH),
South Africa have yielded rich fossil assemblages of late Pliocene to
early Pleistocene age, which include a range of hominin species (A. africanus, A. prometheus, A. sediba, P. robustus, H. ergaster, H. naledi and early Homo) and associated mammals, reptiles, and birds (e.g., Vrba, 1975, 1995; Brain, 1993; Tobias, 2000; Berger et al., 2010, 2015).
For the past 3 million years, hominin-bearing deposits in caves formed
in broadly similar settings, involving debris cone accumulations near
cave openings (Partridge, 1973; Wilkinson, 1985; Brain, 1993; Pickering et al., 2007; de Ruiter et al., 2009; Dirks and Berger, 2013; Herries and Adams, 2013; Dirks et al., 2010, 2016b; Bruxelles et al., 2014; Stratford et al., 2014), with deposits cemented by carbonate-rich waters dripping from cave ceilings (e.g., Wilkinson, 1985; Pickering et al., 2011b). In contrast to all other hominin deposits in the CoH, the deposits that host H. naledi
in Rising Star Cave are composed of largely unconsolidated, mud-clast
breccia in a mud matrix with no evidence of coarse clastic sediment
being carried in by water flow. This suggests a different depositional
regime and timing for the sediments and the fossils (Dirks et al., 2015, Dirks et al., 2016a).
Rising Star Cave is situated in the Bloubank River
valley, 2.2 km W of Sterkfontein Cave. The cave system comprises several
kilometres of mapped passageways (Figure 1a)
that are stratigraphically bound to a 20–30 m-thick, chert-poor
dolomite horizon capped by a 1–1.3 m-thick chert unit that forms the
roof to the cave system (Dirks et al., 2015).
Geological mapping and laser-theodolite surveys indicate that this roof
is intact and not penetrated by significant shafts that open to surface
(Dirks et al., 2015; Kruger et al., 2016). The broader geological setting of the cave is discussed in Dirks et al. (2015), (Dirks et al., 2016a).
Figure 1
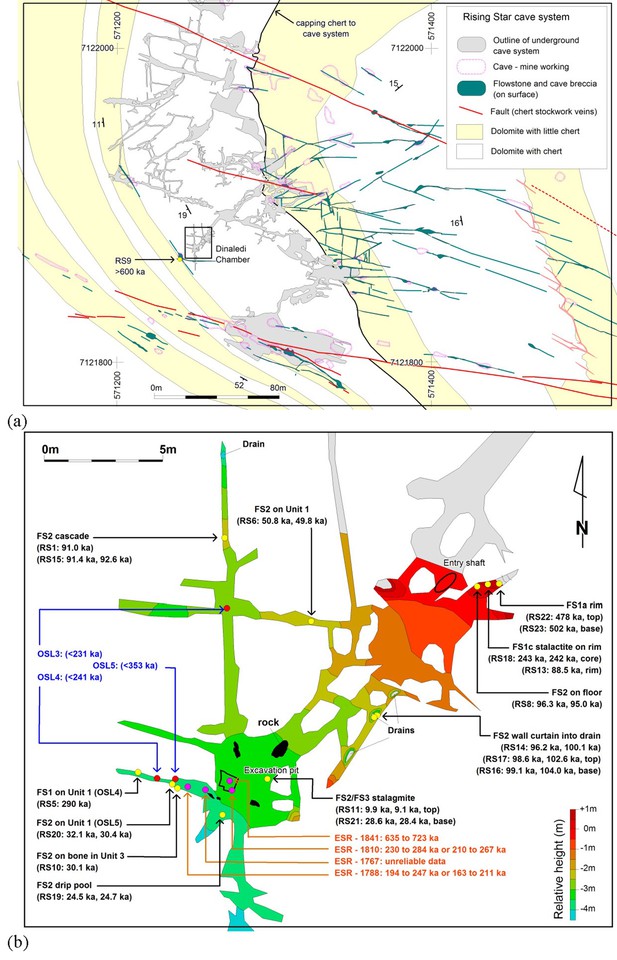
Location of Rising Star Cave and the Dinaledi Chamber.
(a) Simplified geological map showing the position of the Rising Star Cave (in grey); (b) close-up map of the Dinaledi Chamber showing the distribution of the dating samples, including: U-Th …
The Dinaledi Chamber, which contains most of the fossils of H. naledi, is ~30 m below surface and ~80 m in a straight line from the nearest present-day opening to the surface (Figure 1a). The main cavity forming the Dinaledi Chamber is ~15 m long with variable widths not exceeding 2.5 meters (Figure 1b), and expands near the intersection with a crosscutting passage, which is the location of the main excavation site to date (Figure 1b).
There is no evidence that the present entrance into the Dinaledi
Chamber has significantly changed since the deposition of the fossil
hominins, with sediment accumulating mostly near the current access
point (Dirks et al., 2015, Dirks et al., 2016a; Figure 2).
Samples for dating were collected from the various flowstone horizons
and stratigraphic units exposed in the Dinaledi Chamber (Figures 1b, 2, 3, 4 and 5) as well as from fossil material itself (Figures 4, 6 and 7).
Figure 2
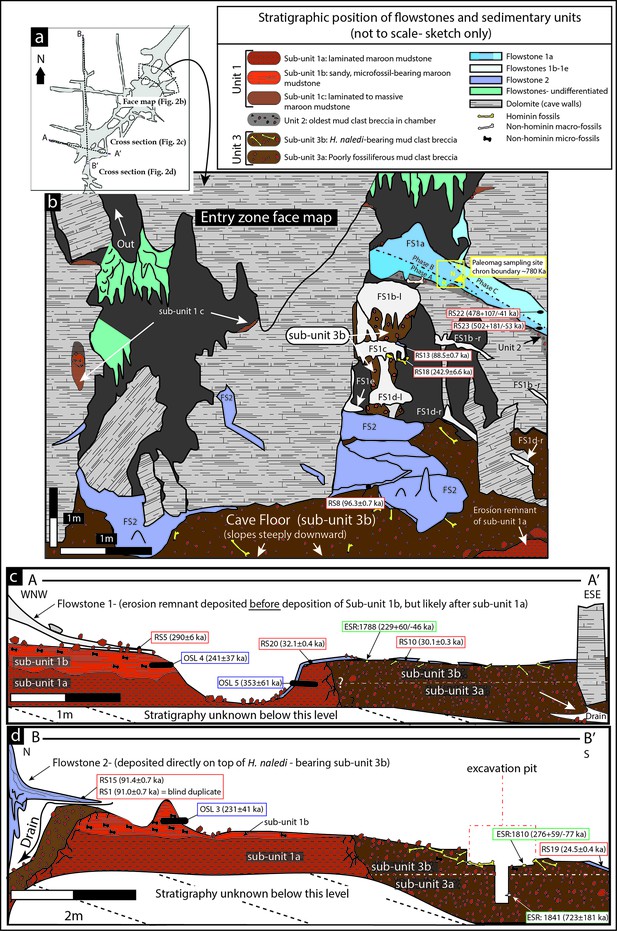
Geological face map and cross-sections through the sediment pile at different locations in the Dinaledi Chamber, illustrating the relationships between the flowstone groups and sedimentary units.
The positions of the section lines are shown in (a); a face map of the entry zone of the Dinaledi Chamber (looking NE) is shown in (b); geological cross-sections through the central part of the …
Lithologic and stratigraphic context for dating
The Dinaledi Chamber contains deposits of
fine-grained, muddy sediments intercalated with flowstone drapes. The
sediments include various types of orange, laminated mudstone and mud
clast breccia distributed across three broad lithostratigraphic units
(Units 1, 2 and 3; Dirks et al., 2015)
that filled parts of the chamber over time. Based on variations in
sediment composition, fossil content and/or stratigraphic position of
each unit, we have divided Unit 1 into sub-units 1a, 1b and 1c, and Unit
3 into sub-units 3a and 3b, to more precisely define the stratigraphic
packages targeted for dating (Figure 2).
The units are separated by erosional unconformities or flowstone
intercalations, but do not all necessarily occur in direct contact with
one another due to the complex nature of caves as depositional systems
(e.g., Brain, 1993; Martini et al., 2003).
In addition, apart from sediments accumulating along the floor of the
cave chamber, sediment in the form of orange mud deposits also
accumulated inside fractures and along ledges higher up in the Dinaledi
Chamber (Figure 2b),
where it formed as a result of the combined effect of in situ
weathering and deposition from water flowing down fractures and side
walls. All units and sub-units are time-transgressive, meaning that they
are lithostratigraphic units and not chronostratigraphic units that
occur in strict temporal order. Periods of sedimentation alternated with
periods of erosion, during which sediments were either redeposited or
removed from the chamber via floor drains, resulting in erosional
remnants of all units occurring in a variety of stratigraphic positions (Dirks et al., 2015).
Stalactites have formed at drip points along the
roof and associated stalagmites formed below these points. In one area
below the entrance to the chamber, these drip points repeatedly formed
flowstone aprons over cave sediments that dip towards the deeper part of
the chamber. Flowstone also formed as cascades and curtains that
developed where water seeped down fractures and ran along the walls to
locally spread out, horizontally, across the sediments comprising the
cave floor (Dirks et al., 2015).
The flowstones have preliminarily been sub-divided into three groups
demarcating semi-contemporaneous generations of formation, which we
named Flowstone Groups 1, 2 and 3 based on their appearance and
relationships with each other, and with the floor sediments and other
litho-stratigraphic units in the chamber. In making this subdivision it
was realised that each group of flowstones will probably comprise a
range of ages representing separate flowstone forming events (Dirks et al., 2015), a fact borne out by the ages presented below (Table 1).
Flowstone Group 1 (FS1 in Table 1; Figures 1b, 2 and 3)
includes remnants of what are interpreted to be generally older
flowstone units that were partly dissolved and resorbed to leave behind
rims or aprons along the side walls of the cave chamber, some with
sediment attached below them. Flowstone remnants interpreted as
Flowstone Group 1 are mostly restricted to five staggered remnants
(Flowstones 1a-e), one above the other in reverse stratigraphic order
(oldest on top, youngest at the bottom), near the entry shaft into the
Dinaledi Chamber (Figure 2b). Flowstone Group 2, the most extensive group of flowstones in the chamber (FS2 in Table 1, and Figures 1b, 2 and 3),
comprises wall aprons and sheets that have spread out across the floor
of the Dinaledi Chamber together with drip pools, cascades, curtains,
stalactites and stalagmites that connect to these sheets, and,
therefore, formed in conjunction with them. Flowstone Group 3 (FS3 in Table 1 and Figures 1b, 2 and 3)
comprises the flowstone deposits that are actively forming below
existing drip points, and include fresh growth of delicate crystals of
aragonite and calcite in floor sediments and along cave walls.
Sedimentary deposits within the Dinaledi Chamber can be organized into three primary stratigraphic units (Dirks et al., 2015). Unit 1
consists of deposits of non-lithified, laminated, orange mud
interpreted as suspension deposits in standing water (Facies 1a of Dirks et al., 2015), and laminated mud with fine sand containing small-scale ripple cross laminations and rodent remains (Facies 1b of Dirks et al., 2015),
reflecting deposition by shallow, flowing water along the cave floor,
with additional sandy material accumulating near local entry points,
where fractures higher in the chamber act as sediment conduits (Dirks et al., 2015, Dirks et al., 2016a).
Within the Dinaledi Chamber Unit 1 deposits
can be divided into three sub-units provisionally called sub-units 1a,
1b and 1c. It is assumed that Unit 1 is time-transgressive and future
work may reveal additional sub-units. Sub-unit 1a is composed of
laminated orange mudstone with isolated lenses of sandy material, occurs
as erosion remnants along the cave floor, and is possibly more
extensive beneath younger deposits in the chamber. Sub-unit 1b is
dominated by sandy orange mud deposits that are rich in micro-faunal
remain, stratigraphically overlies deposits of sub-unit 1a (Figure 2c and d),
and may have formed through the partial erosion and re-deposition of
sub-unit 1a. Deposits of sub-unit 1c are similar in appearance and
composition to the laminated, muddy sediments of sub-unit 1a, but they
occur along chert ledges, solution pockets and fractures in the chamber
walls and along the entry shaft, higher up in the cave chamber (Figure 2).
The orange mud is mostly the product of the cave formation process,
representing the insoluble residue left over when cavities develop via
dissolution of dolomite (Dirks et al., 2015).
Some of the mud-bearing waters seeping out of the fractures would have
flowed as water films along the cave walls to deposit mud on ledges and
in fractures to form sub-unit 1c, whilst elsewhere this water would have
dripped to the floor to contribute to the deposition of sub-unit 1a and
1b.
Unit 2 is composed of largely lithified mud
clast breccia consisting of angular to sub-angular clasts of laminated
orange mudstone (similar to that found in Unit 1), embedded in a brown
mud matrix (Facies 2 of Dirks et al., 2015).
The mud clasts are interpreted to be derived locally due to wetting and
drying of orange mud deposits, which led to auto-brecciation, and
subsequent erosion and re-deposition of angular mud clasts (Dirks et al., 2015).
We hypothesize that the mud clasts forming Unit 2 are partly derived
from erosion of deposits of sub-unit 1c, and partly from a yet
unidentified unit that was likely deposited in fractures within and
above the chamber entry zone. Two macro-fossils (partial shafts of long
bones) that are non-specific, but not hominin, have been found in Unit
2.
Unit 2 sediments are only exposed as hanging
remnants attached below the remains of a composite flowstone sheet
(Flowstone 1a) near the entrance shaft into the chamber (Figure 2b; Dirks et al., 2015). Note that in Dirks et al. (2015)
Unit 2 was originally defined to also include sediments below
Flowstones 1b-e; however, based on our new dating results, the revised
definition of Unit 2 has been narrowed to only include the more
indurated and distinctly darker coloured erosional remnants of mud clast
breccia under Flowstone 1a, which are notable for their absence of
hominin fossils. Unit 2 sediments accumulated as a sloping debris cone
of mud clast breccia below a vertical fracture system before being
covered by flowstone (Flowstone 1a). The debris cone of mud clast
breccia was subsequently eroded leaving behind hanging erosion remnants
of Unit 2 below a flowstone apron (Figures 2b and 3l).
The processes that caused erosion of the Unit 2 debris cone led to the
deposition of Unit 3 sediment along the floor of the Dinaledi Chamber as
shown in Figure 8.
Unit 3 is composed of largely unlithified, clast-supported, mud clast breccia (Facies 2 of Dirks et al., 2015),
dominated by reworked angular to sub-angular mud clasts, which are
interpreted as being locally derived from the reworking of Units 1 and
2. Unit 3 accumulated below the hanging remnants of the Unit 2 debris
cone near the entry shaft, and also extends along the current, sloping
cave floor to the SW end of the chamber (Figures 2c and 8).
Unit 3 sediments are dynamic in the sense that they are poorly
lithified in most places and actively slump towards, and erode into,
floor drains that occur in parts of the chamber where sediment is being
washed down to deeper levels in the cave (likely as a result of
fluctuations in the ground water level). Remains of Unit 3 sediment are
attached to apron-like erosional remnants of Flowstones 1b-e near the
entrance shaft (Figure 2a and 3l). Erosional remnants of Unit 3 under Flowstone 1c contain in situ long bones consistent with H. naledi, which are actively eroding out and accumulating along the present cave floor. Note that Dirks et al. (2015)
originally included these erosional remnants as part of Unit 2.
Everywhere else, Unit 3 deposits are spread across the cave floor as
loosely packed, semi-moist, orange mud clasts of varying sizes in which
bone material of H. naledi is distributed. Unit 3 is partly covered by sheets of Flowstone Groups 1, 2 and 3.
Unit 3 has been divided into a lower and an upper sub-unit, termed sub-unit 3a and 3b (Figure 2),
based on the respective absence or presence of hominin fossils.
Sediments belonging to sub-unit 3a are not directly exposed in the
chamber, but their presence has been confirmed in the deepest part of
the excavation area (Figure 2d).
In contrast sub-unit 3b is exposed within the talus cone near the entry
shaft and along the cave floor, and contains all of the known H. naledi fossils in the chamber (Figure 2c and d). The thickness of sub-unit 3b is thought to be no more than 20–30 cm (see below).
The distribution of fossils, units, and flowstones
All hominin bones identified in the Dinaledi Chamber are contained in deposits of sub-unit 3b. Bones attributed to H. naledi
have been recovered as: (a) isolated elements that weathered out from
erosion remnants of sub-unit 3b below Flowstones 1b-e; (b) as fragmented
remains scattered across the cave floor; and (c) as partly articulated
remains from a single excavation pit down to a depth of ~20 cm below the
current floor level (Dirks et al., 2015).
Preliminary ground penetrating radar work (Naidoo, 2016)
suggests that Unit 3 deposits along the floor of the Dinaledi Chamber
could be up to 1.5 m thick. A 50 cm-deep sondage was dug in the centre
of the excavation pit, which itself is 20 cm deep, to indicate a minimum
depth of 70 cm for the mud clast breccia pile of Unit 3. The top 20 cm
of this sediment contains H. naledi remains and is part of sub-unit 3b (Figure 2d).
A discrete contact occurs at 15–20 cm depth, below which no more
fossils were encountered with the exception of a single juvenile baboon
tooth (sample 1841; Figure 7) that was recovered from a depth of 55–60 cm below the original cave floor surface in sediment of sub-unit 3a (Figures 2 and 8).
Staining patterns on bone fragments, skeletal
element representation, and the fact that bones can be seen to weather
out from erosional remnants of sub-unit 3b, indicate that part of the
fossil assemblage has been reworked (Dirks et al., 2015).
The presence of well-articulated remains in the excavation pit away
from the chamber entrance indicates that some of the remains entered the
cave intact. The mixed taphonomic signature suggests that fossils
entered the cave over a period of time, which is minimally assumed to be
during deposition of sub-unit 3b, and before deposition of Flowstone
1c. Fossil entry may have continued as sediment accumulations of
sub-unit 3b near the entry shaft were reworked and redistributed along
the cave floor (Figure 8).
The stratigraphic relationships in the Dinaledi
Chamber suggest that Unit 1 sediments were deposited over a long period,
which both predates and spans the more limited depositional timeframes
of Units 2 and 3. Hence, Unit 1 is time-transgressive, meaning that
these sediments were (and are) constantly forming in different parts of
the chamber due to weathering of the dolomitic cave walls (i.e., wad
formation sensu Martini et al., 2003),
and that their age is dependent upon where in the cave the material is
located. At present, we can only divide Unit 1 into three sub-units, but
we hypothesize that an older sub-unit consisting of laminated orange
mudstone exists (or existed) higher up in the chamber as well (possibly
only on ledges and in fractures), which was eroded to provide some of
the sediment that formed Unit 2 and parts of Unit 3, near the entry
shaft.
Flowstone 1a, which overlies remnants of Unit 2, is
the oldest flowstone unit in the chamber, and displays evidence of
multiple phases of flowstone formation followed by partial dissolution (Figure 2b).
Flowstone dissolution occurred during time periods when the water table
was elevated and the chamber was filled with standing water. The
erosion remnants of Flowstone 1a dip towards the deeper part of the
chamber, indicating that at the time of its formation, a sloping debris
cone of Unit 2 sediment was present. Erosion of Unit 2 sediments from
underneath Flowstone 1a only occurred after the flowstone had formed and
lithified the top of Unit 2. Following erosion of Unit 2, deposition of
Unit 3 began, as sediment and mud clasts spread out over the cave floor
and also filled much of the space underneath Flowstone 1a. This has led
to an inverted stratigraphy near the cave entrance, although a normal
stratigraphy is documented at the bottom of the chamber, where the cave
floor is flat lying and sediment of Unit 3 progressively built up (Figures 2b and 8). At some point during these processes remains of H. naledi
entered the cave chamber, marking the start of deposition of sub-unit
3b. Following deposition of sub-unit 3b and the hominin remains,
Flowstones 1b-e were deposited over sub-unit 3b in the entry zone. These
flowstones have been interpreted as younger than Flowstone 1a, but
older than the Flowstone Group 2 sheets along the cave floor. In other
words, after deposition of Unit 3 commenced to form the talus cone near
the entrance of the chamber, parts of the cone slumped and eroded down
towards deeper parts of the chamber after Flowstones 1b-e were
deposited, but before Flowstone Group 2 was deposited. This slumping
motion was probably driven by sediment being removed from the base of
the stratigraphic pile through floor drains.
Flowstone Group 2 covers erosion remnants of
Flowstones 1a-e as coatings and stalactites along drip rims. In places,
Flowstone Group 2 also covers erosion remnants of Unit 1 and Unit 3
along the floor and displays variable relationships with Unit 3 (Figure 2).
Where parts of Unit 3 have been eroded via floor drains, hanging
remnants of Flowstone Group 2 can be found attached to the walls as
fringing aprons, up to 10 cm above the current floor level, establishing
the fact that parts of the floor are currently in a state of erosion.
In other places, Flowstone Group 2 sheets directly overlie Unit 3 and
the H. naledi fossils it contains. These varying relationships
indicate that Flowstone Group 2 sheets were deposited over an extended
period of time, post-dating deposition and partial reworking of sub-unit
3b.
In summary, the stratigraphic context indicates that the H. naledi
fossils entered the cave during deposition (and possibly during partial
reworking) of sub-unit 3b, after deposition of the older sediments of
Unit 1 (sub-unit 1a) and Unit 2. Several isolated, non-hominin bone
fragments in hanging erosion remnants of Unit 2 and a single baboon
tooth in floor sediments in sub-unit 3a were deposited prior to the
entry of the hominin remains. The accumulation of Unit 3 along the cave
floor involved a dynamic interplay between the accumulation of mud clast
breccia below sediment entry points or in situ sediment sources (Unit 1
and Unit 2) in the chamber, and erosion through floor drains resulting
in contrasting stratigraphic relationships across the chamber (Figures 2 and 8).
Dating the H. naledi fossils
Most fossil deposits in the Cradle of
Humankind that have been dated are between 0.5 and 3.7 Ma old and
consist of bone material encased in well-cemented hard clastic rocks
commonly referred to as cave breccia (e.g., Wilkinson, 1985; O'Regan and Reynolds, 2009; Herries et al., 2009; Pickering et al., 2011b; Granger et al., 2015).
In the absence of volcanic deposits, it is generally difficult to
obtain accurate ages for the fossils, not just because reliable
techniques are few, but mostly because the stratigraphic sequences in
the caves are complex, discontinuous and frequently reworked (e.g., Brain, 1993; Pickering et al., 2011a; Bruxelles et al., 2014; Stratford et al., 2014).
Workers have relied on a combination of biochronology of faunal
remains, palaeomagnetic work and a range of radiometric methods,
including U-Pb, U-Th and ESR dating targeting flowstones and fossil
teeth (e.g., Vrba, 1975; Partridge et al., 1999; Berger et al., 2002; Walker et al., 2006; Herries et al., 2006, 2013, 2014; Herries and Shaw, 2011; Dirks et al., 2010; Pickering and Kramers, 2010; Pickering et al., 2011a; Herries and Adams, 2013), as well as limited cosmogenic (10Be, 16Al) dating (e.g., Partridge et al., 2003; Granger et al., 2015; Dirks et al., 2016b). Whilst some of these techniques are well established, others such as the application of cosmogenic isochrons (e.g., Granger et al., 2015) are relatively new and not without significant analytical (and interpretative) challenges (Kramers and Dirks, 2017), and all efforts are strongly dependent on the stratigraphic interpretation of the fossils or units that are being dated.
Unlike other fossil deposits in the Cradle of
Humankind, the remains in the Dinaledi Chamber are largely restricted to
hominins. This makes it impossible to use biochronology as a
preliminary technique to assess the age of the fossils. In addition, the
fossils are contained in mostly unconsolidated muddy sediment with
clear evidence of a mixed taphonomic signature indicative of repeated
cycles of reworking and more than one episode of primary deposition (Dirks et al., 2015). This indicates that caution is required when interpreting the stratigraphy and the age of the fossils they contain.
In preparation for this study, trial dating of the
deposits in the Dinaledi Chamber was undertaken to obtain an indication
of the age of the deposit and the best techniques to apply. Preliminary
work was focussed on assessing the viability of U-series techniques for
flowstone dating, using 14C for dating bone fragments, and using OSL to test samples of quartz-bearing Unit 1 (Dirks et al., 2015).
Initial tests were carried out at the University of Johannesburg (UJ)
to assess suitability for U-Pb dating, which allows for the dating of
older (>500 ka) flowstone material (e.g., Walker et al., 2006; Pickering et al., 2010; Pickering and Kramers, 2010), on the assumption that the H. naledi material could be older than 1 Ma based on its primitive morphology (Berger et al., 2015; Dembo et al., 2016; Hawks and Berger, 2016; Thackeray, 2016; Hawks et al., 2017).
It was found that the older flowstones in the Dinaledi Chamber
contained excessive common Pb caused by the inclusion of detrital
material (mainly clays) making them unsuitable for U-Pb dating (Dirks et al., 2015).
In contrast, preliminary tests with U-Th disequilibrium dating at James
Cook University (JCU) returned promising results. U-Th dating is more
precise in the <500 a="" affected="" and="" anticipated="" be="" by="" critically="" data-behaviour-initialised="true" dating="" detrital="" disequilibrium="" e.g.="" fossils="" href="https://elifesciences.org/articles/24231#bib14" initial="" is="" ka="" less="" material.="" may="" much="" originally="" range="" revealed="" tests="" than="" that="" the="" u-pb="" u-th="" with="" younger="">Dembo et al., 2016500>
Preliminary tests with OSL were conducted at the
University of the Witwatersrand (Wits) on samples from Unit 1, which
were assumed to be older than the fossils of H. naledi. These
preliminary studies, and the results contained in this paper, are the
first OSL results for cave sediments from the CoH, and again indicated
that the H. naledi fossils were probably relatively young (i.e.,<500 ka="" p="">
Tests with radiocarbon (14C) dating were
undertaken through a commercial facility (Beta Analytic Inc. in
Florida), to ensure a fast turn-around time for results. At the time
these dating tests were done, it was already known from U-Th and OSL
tests that the H. naledi fossils would be too old to be dated by 14C.
Nevertheless, analyses were carried out as part of the due diligence
process, and the results of these tests are presented here. Following
this initial work, no further radiocarbon studies were carried out.
The preliminary results have guided the subsequent
dating strategy and sampling approach reported here. The dating strategy
was designed to achieve three objectives: (i) establish a detailed
stratigraphy for the cave sediments in the Dinaledi Chamber; (ii) date
sedimentary units that potentially bracket the fossil-bearing deposits;
and (iii) date the fossils directly.
To obtain an upper age limit for the fossil-bearing
deposits of Unit 3 (i.e. sub-unit 3b), we conducted U-Th dating of
flowstones that directly overlie Unit 3. A large number of such
flowstones were sampled with the aim of finding the oldest flowstone
directly overlying H. naledi fossils. To obtain a lower age limit
for sub-unit 3b, erosional remnants of Unit 1 sediments that were at
least partially covered by fossil-bearing sub-unit 3b sediments, were
sampled for OSL dating on the assumption that sub-units 1a and 1b in
these areas are older than sub-unit 3b (Dirks et al., 2015). This was done in the full knowledge that OSL dating of cave sediments is complex and difficult to interpret (e.g., Roberts et al., 2009),
and probably imprecise. As an internal control, we also sampled
flowstones that cover the outcrops of sub-units 1a and 1b from which OSL
samples were taken. These flowstones were dated with U-Th with the
expectation that they are younger than the underlying Unit 1 sediments.
In addition to OSL, Flowstone 1a, which overlies Unit 2 sediments, was
sampled for palaeomagnetic analyses. This flowstone was targeted,
because it was expected to be the oldest flowstone in the chamber and
possibly older than 780 ka, and hence could potentially record reverse
magnetic polarity (e.g., Singer, 2014). In this case, this would constrain the minimum age of Unit 2.
The best age estimates for H. naledi can be obtained by directly dating fossil material. It was clear from preliminary tests that this could not be achieved with 14C, and instead combined ESR and U-Th disequilibrium dating techniques (US-ESR; Grün et al., 1988) were applied to three H. naledi teeth that were freshly collected from near the site of the original excavation (Figures 1, 2, 4 and 6), as well as a single baboon tooth (cf. Papio) that had been recovered from sub-unit 3a below the hominin-bearing horizon (Figures 2d and 7).
Once results were obtained for ESR and U-Th dating,
it became apparent that OSL dating would only provide general age
constraints that confirmed the ESR results, but in their own right did
not return additional age constraints for the fossils. OSL results were
also difficult to interpret in the complex cave environment that was
strongly affected by Rn loss (see Discussion). It was, therefore,
decided not to pursue more detailed OSL studies at this stage, even
though we did carry out preliminary tests for single grain and feldspar
analyses at the University of Wollongong, to assess the suitability of
these techniques. Pilot results are encouraging, and suggest that
future, detailed OSL studies are worth pursuing.
500>
Nenhum comentário:
Postar um comentário
Observação: somente um membro deste blog pode postar um comentário.